1. Introduction
Globally there is unprecedented extraction of natural sand (Prakash Chandar and Loganathan, 2022; Susheel Kumar et al., 2022); especially along river beds (Pandya et al., 2023) and marine coastal zones with heavy mineral-rich sands (Prasad et al., 2020; Sundararajan et al., 2021). According to Szabo and Hedge (2021) and Singh et al. (2022), sand is currently the 2nd most consumed natural resource after water. The increase in the demand for sand has mainly been due to infrastructural development as sand (river sand) is crucial for construction (Devi et al., 2023) and the manufacture of concrete materials e.g. concrete bricks (Susheel Kumar et al., 2022) to satisfy the increasing urbanization (Murugesan et al., 2020) due to the increasing population (Sathya et al., 2023). Globally, it is estimated that about 40 (Bhatawdekar et al., 2022) and 47–59 billion tonnes (Szabo and Hedge, 2021) of natural river sand are demanded annually, and projections indicate that the demand will increase to about 86 Gt (Gigatons) by 2060 (OECD, 2019); surpassing the rate at which sand replenishes (Lamb et al., 2019; Marschke and Rousseau, 2022). Today, sand accounts for about 85 percent of the annual volume of minerals mined globally (Filho et al., 2021). A number of studies document that sand mining would not have been a problem per se as sand satisfies the global construction demands (Torres et al., 2017) and marine sand is crucially helping in developing coastal defenses and infrastructure that help mitigate climate change-induced shocks e.g. coastal floods and storm waves (Hübler et al., 2021; Matovu et al., 2022). However, there is increasing evidence of the dangers of sand mining especially along environmentally sensitive zones that affect ecosystems and livelihoods (IRP; 2020, 2021).
A number of reports have critically examined the detrimental consequences of sand mining on biodiversity and livelihoods, including resource user conflicts, coastal over-urbanization thus loss of recreation values, pollution, and human-induced climate change (Fletcher et al., 2021; WOA III, 2023). Sand mining has further led to increased transboundary crime especially in the global south e.g. in India where sand mining activities are run by sand mafias (Mahadevan, 2019). This is worsened by the fact that the sand trade is one of the less regulated activities globally (Chatham House Database, 2023) and the lack of comprehensive global frameworks for sand mining and trade (IRP, 2020). According to the HS4 Product Classification, just 2.9% of sand trade was subject to tariffs by 2020 (OEC, 2023). This poses a problem for implementing Agenda 2030, which asks for the inclusive and integrated development of strategies for the social, economic, and envi-ronmental management of coastal resources (World Bank, 2020). This perspective documents that sand mining is a critical sector that could scupper the Blue Economy (BE) Agenda advanced at Rio +12 as it is threatening to BE resources and sectors that most people and economies; especially in the developing world depend on e.g. coastal fishing and tourism (UNCTAD, 2021). In addition, few studies especially in the Global South put emphasis on documenting the complexities of sand mining for fear of reprimand from local authorities, forceful eviction from land or violence against disgruntled voices (Mahadevan, 2019).
2. Sand Mining in India
To put this into perspective, our study shall use a case study of India to document the complexity of sand mining and trade and its effects on livelihoods, ecosystems, and the implications for sustainable development. Since the 1990s, sand has become a scarce but crucial natural resource in India (India Rivers Forum Report, 2020). Today, most of the sand is either extracted along river beds (Padmalal and Maya, 2014a) or along marine coasts (Bisht, 2019; Parthasarathy and Usha, 2014) e.g. Tuticorin in Tamil Nadu (Cherian et al., 2011) that contain valuable placer minerals e.g. monazite-8 million tonnes (71 percentage of global resources), ilmenite-estimated at 340 million tonnes, rutile, sillimanite-130 million tonnes, garnet-estimated at 107 million ton, zircon-estimated at 21 million tonnes (Sundararajan et al., 2021; Thakur et al., 2014) and rare earth minerals e.g. titanium e.g. in Tamil Nadu (Bisht, 2019), and lithium in black sands of Chavara in Kerala (Prasad et al., 2016, 2020). In fact, most reports have indicated an increase in the river and marine sand demand and supply in most coastal states of India (India Rivers Forum, 2020) (Figure 1). In addition, the local annual demand for river sand is estimated at 60 million metric tons (Singh et al., 2022).
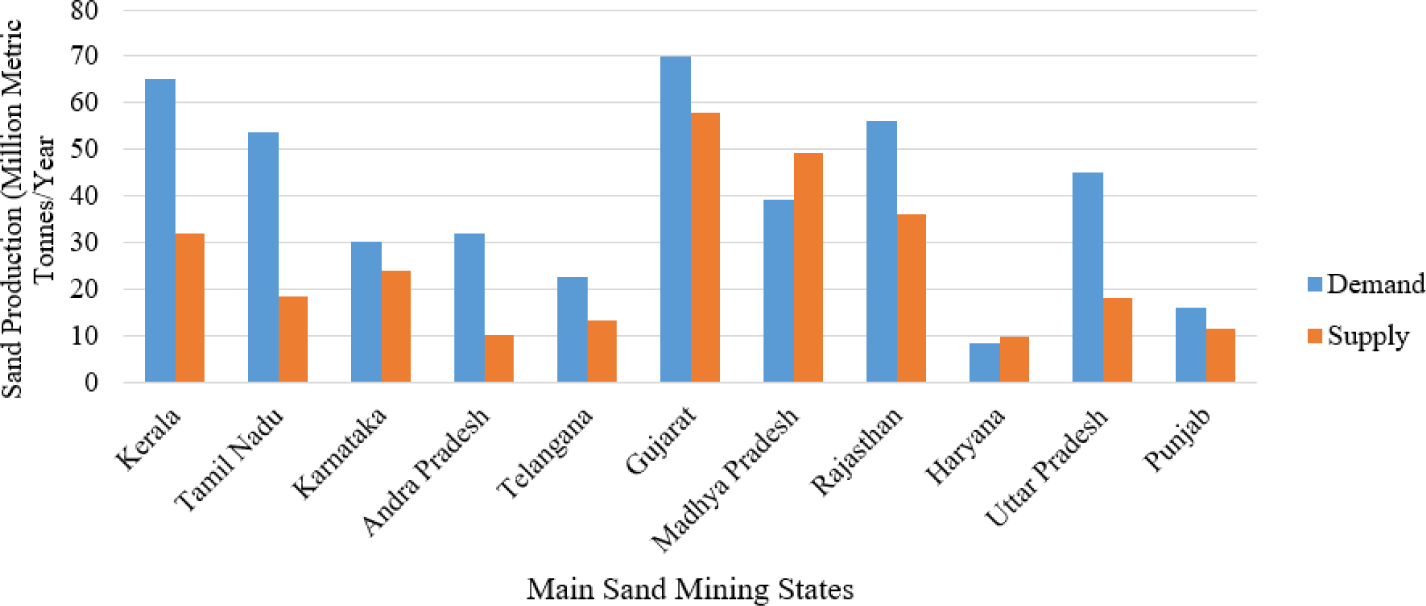
The increase in India’s share in the global sand trade is also evident (Singh et al., 2022). According to the 2023 Earth Trade Database, as of 2020, India accounted for 1.8 percent of the world’s sand and gravel commerce, or 96.7 million USD. After China and the USA, India is currently one of the top consumers of building sand (Mahadevan, 2019; National Geographic Report, 2021). From 2000 to 2017, the demand for sand in India tripled, generating a market with a value of 150 billion rupees, or slightly more than two billion USD (Mahadevan, 2019). Over 35 million people are employed in the sand mining industry, which is thought to contribute 9% of India’s two trillion dollar economy. According to additional estimates, India’s sand demand will reach over 1.5 billion tonnes by 2030 (Kukreti, 2017). The increase in sand demand has been a result of population increase e.g. in metropolises such as Mumbai where the daily demand for river sand is estimated at 1 kilogram (Singh et al., 2022), and increased affluence e.g. in Kerala (Mahadevan, 2019) which has ballooned the demand for housing facilities in urban areas (Bisht, 2019) and infrastructural development projects (Prasad et al., 2020). In fact, India’s sand demand surpasses the natural replenishment and supply rates (Singh et al., 2022; Talukdar and Das, 2021). A study by Ramya and Nivetha Kumari (2020) clearly puts this in perspective that in every 30 miles distance where there is a building site in India, there is ongoing sand mining. This demonstrates that the availability of sand is key to the development of India’s infrastructural projects (Nithya et al., 2017) e.g. port facilities in Tuticorin (Singh et al., 2022) and urban infrastructure concrete demands e.g. in Srirangam Island (Muthamilselvan et al., 2016) estimated at 450 million cubic meters annually (Nithya et al., 2017).
Though this is commendable to socio-economic development (Rama-chandra et al., 2017), there is growing evidence of negative externalities of sand mining in most coastal zones e.g. around the Gulf of Mannar (Jayaprakash et al., 2016) and ecologically fragile zones (Devi et al., 2023); including river banks e.g. Ganges (Saha, 2016), backwaters e.g. in Chavara (Prasad et al., 2020), and other areas rich in biodiversity thus affecting sustainable development (Ghosh et al., 2016). According to estimates by Sreedharan and Padmalal (2011), the rate of sand extraction from some rivers in Kerala has been 40 times higher than the rate of replenishment. According to Beiser (2015), the daily production of illicit sand from a single site appears to vary between 150 and 500 lorry loads, and the costs of increased mining in terms of the environment, society, and economy have multiplied (Padmalal and Maya, 2014b). In Tamil Nadu, the loss of revenue from riverine and coastal sand mining is estimated to be 2.7 billion USD and 4.1 billion USD, respectively (Mahadevan, 2019), and in Kerala, sand mining has been linked to environmental calamities like the 2018 floods (Datta, 2018). This is worsened by the lack of or inadequate sand regulations in most coastal states (India Rivers’ Forum, 2020) and limited scholarship to specifically correlate the effects of sand mining on the sustainable development and livelihoods of vulnerable people (Talukdar and Das, 2022). We thus argue that the increase in less scientific sand mining processes regulated by the sand mafia, which is increasingly becoming a transboundary and transnational enterprise (Mahadevan, 2019) is likely to increase the vulnerability of coastal livelihoods; threaten sustainable ecosystem mana-gement and scupper governance mechanisms to regulate the sand mining in India (Bisht and Gerber, 2017). This complex scenario forms the basis of this study which aims at documenting the effects of sand mining in India and what are the likely implications to sustainable development.
3. Materials and Methods
The study used a systematic literature review approach to access and obtain data and information on the effects of sand mining on coastal livelihoods, development, and governance in India. According to Neumann (2014), systematic literature reviews have recently grown in popularity across a variety of disciplines, particularly social science and multidisciplinary research, and are increasingly recognized as an objective and replicable strategy for conducting reviews on a specific research topic (Ferrari, 2015). Unlike conventional reviews, systematic literature reviews adhere to a set of predetermined guidelines known as the preferred reporting items for systematic reviews and meta-analyses method (PRISMA) to identify, include, exclude, extract, and interpret results in accordance with the study objectives (Bahinipati et al., 2021; Neumann, 2014).
The first step to extract data involved keyword searches in Scopus electronic databases in June 2023 (Table 1) and 179 results, which were all saved as Scopus.ris files to be considered for screening.
Since we intended to use the PRISMA review framework, we further used multi-step criteria to screen (Neumann, 2014) the 179 extracted results. We imported the extracted results into Covidence screening software. Covidence software is regarded as one of the best tools to conduct a thorough abstract and full-text reading of articles/documents to be considered in the study (Covidence, 2023), relating to the research topic and objectives. After conducting full text and abstract reading of the documents, 85 studies were included for review in the study (Figure 2).
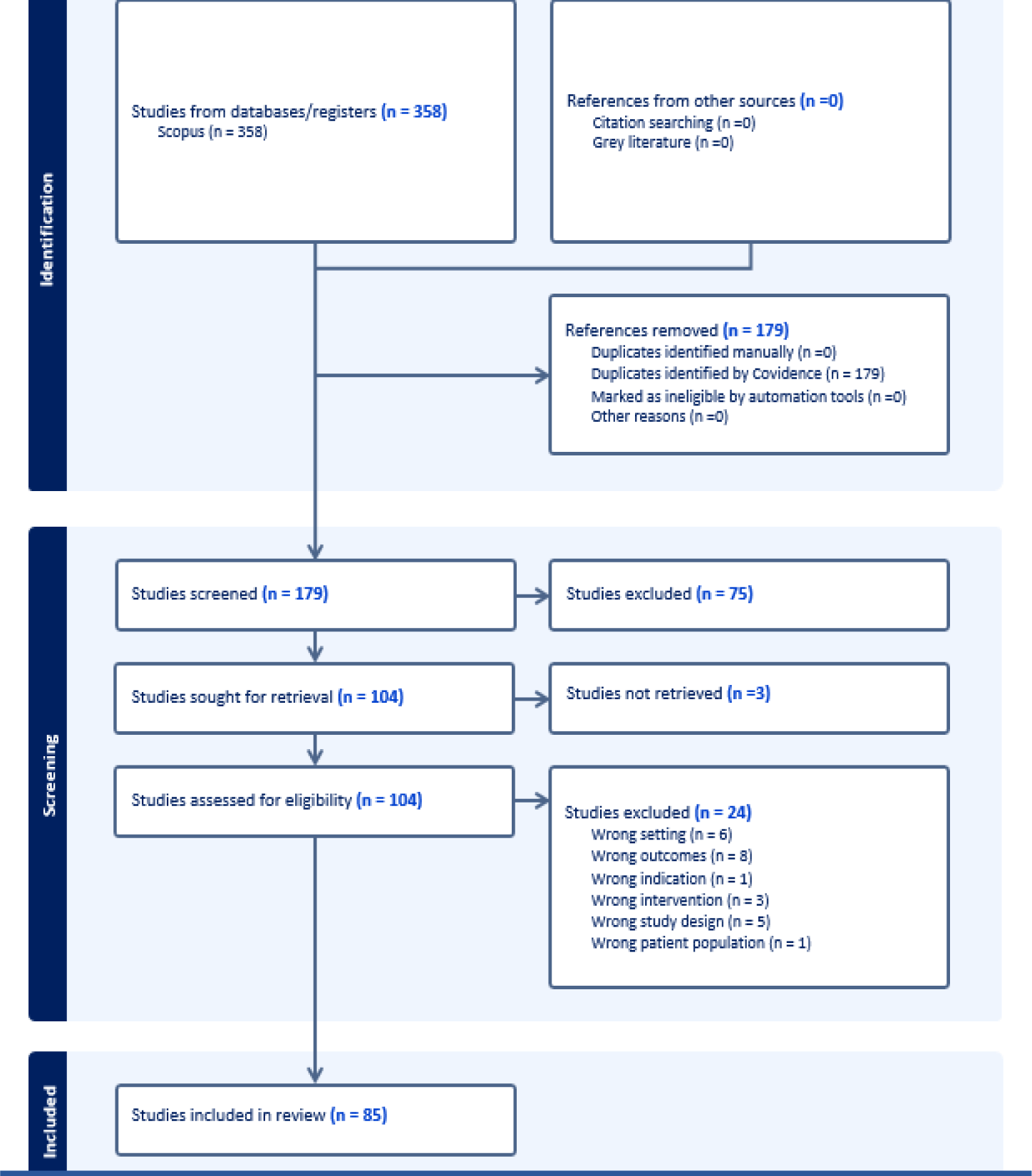
To easily extract the main effects of sand mining in coastal regions, we imported the articles into an Excel sheet. For each article, a specific breakdown was made to identify the area of focus for the article e.g. a coastal state, river system/catchment, or local area e.g. district where sand mining takes place, the effects on ecosystems, and the effects on livelihoods. Depending on the nature of the effect, we further related it to a Sustainable Development Goals (SDG) (UN-SDG, 2023) or SDG indicator or governance challenge it creates e.g. in the coastal zone or livelihood or ecological management in the context of India and globally.
4. Results and Discussion
In this section, we explain the impacts of sand mining on both livelihoods and the environment and how the impacts could scupper the attainment of sustainable development. For easy comprehension, we breakdown the implications as (i) implications on the environment and (ii) implications on livelihood. The identification of these implications would help in reflecting on the paradox that countries e.g. India face in the context of attaining sustainable goals and in the resource nexus governance (Matovu et al., 2022).
5. Implications on the Environment
In this section, the focus was to identify and explain the impacts of sand mining on the environment. We sub-categorized these impacts into three i.e. on the riverine environment (along or within river channels), on the marine/coastal environment, and on ecosystems/biodiversity (Table 2).
Reviewed documents revealed that the dominant impact of sand mining has been the alteration of the river channels (morphology) thus leading to several channel effects e.g. the 2018 floods along the Mayurakshi River in Jharkhand (Islam et al., 2022). Massive channel shifts have been reported along the Subarnarekha River with riverbank/bank-line shifting of 0.121m in the estuary section and 0.002 m in the upper sections thus increasing flood hazard (Jana, 2021). Related research along the Kangsabati River in South Bengal revealed that maximum thalweg shifting along the Mohanpur 3 (543 m) and Kapastikri 1SS (152 m) with an average rate of 0.66 km from 2002 to 2016 has increased river channel incision in sandchar sites (Bhattacharya et al., 2019c). Similar effects of the shifting of Thalweg and instability of river bars e.g. along the Sali River left bank channel between Sillaghat and Bikrampur in the Damodar have been reported thus erosion of riverbank farmlands (Ghosh et al., 2016). Increased flood risks have also been reported in the Lower Damodar River (Bhattacharya and Das Chatterjee, 2021) and sedimentation of the plains of River Kangsabati especially during the post-monsoon period (Bhattacharya et al., 2016). Similar findings of flood vulnerability were reported in Udupi in Karnataka (Szabo and Hegde, 2021). Devastating impacts of flooding that reduces alluvial sediments have also been reported in major rivers in Kerala where 68% (i.e. 5.968 million ty-1) is lost from the Periyar river, 21% from R. Muvattupuzha (1.864 million ty-1) and 11% from Chalakudy (11%; 0.933 million ty-1) (Sreebha and Padmalal, 2011).
In addition, increased morphological, hydrological, and environmental effects have been reported in the Dooars region of West Bengal e.g. along the Raidak-II River, consisting of 28 mining sites in its course from Bhutanghat to Binnagurialong the river profile that has increased slides at the foothills of Himalayas (Talukdar and Das, 2021) and along Chalakudy, Periyar and Muvattupuzha rivers, in Kerala (Sreebha and Padmalal, 2011). In addition, along the Bhagirathi-Hooghly River in West Bengal, illegal sand mining activities since the 1980s, reduced river channel width by up to 55% thus increasing monsoon flood risks and rejuvenation in meandering streams thus accelerating erosion e.g. along the Navadwip–Kalyani stretch (Ghosh et al., 2020). These have further led to river cave-in as evidenced in a study along the Chandernagore zone in Hooghly along the Ganges Delta where the river has caved in by 400 m in the lower course (Saha, 2015). It is thus evident that the increased channelization accelerates riverbanks erosion and leads to a multitude of river banks effects both on livelihoods and ecosystems.
Recent studies in along river sand mining zones have documented that many rivers and their resources have been decimated and degraded e.g. riverbanks ecosystems and systems (Nithya et al., 2017) e.g. of the Chaliyar River in Kerala (Sathya et al., 2023 ) and in Tamil Nadu (Murugesan et al., 2020). Similar findings have been observed along the Bharathapuzha river in Palakkad and Trivandrum district sections where most natural resources including sand have been depleted (Anand and Reddy, 2014) partly due to increased indiscriminate scooping in floodplains/ It is estimated that small rivers have lost about 10,000 km2 of their catchment area (Padmalal and Maya, 2014c). The decimation of rivers especially via drilling has led to the lowering of groundwater table (Sathya et al., 2023). Related findings were observed in a study along Kangsabati River in South Bengal that reported that the water table reduced e.g. reported at 4.49 m (Mohanpur 3 SS), 3.2 m (Lalgarh 3 SS) and 1.86 m (Kapastikri 2) sections between 2014–2019 due to pit mining and bar skimming (Bhattacharya et al., 2019b). For instance, a study conducted covering 41 rivers in Kerala found that most riverbeds in their storage zones experience a water level lowering rate of 5–20 cm per year (Padmalal and Maya, 2014d) e.g. along R. Ithikkara (Sheeba, 2009).
In addition, this impact has further reduced groundwater quality and salinity due to high levels of silicate weathering and creation of sinkholes (Singh and Dhar, 1997) e.g. samples from a study along River Ganga on the Ajay-Mayurakshi section reported high salinity levels belonging to the C2 (70%, n=77) followed by the C3 category (29.09%, n=32) signifying higher EC and salinity hazard (Sarkar and Islam, 2021). A related study in Tamil Nadu and Chavara in Kerala reported increased levels of groundwater pollution e.g. in the Kanyakumari district with most groundwater having 39% of total heavy mineral content (Sundararajan et al., 2021). Similar observations on increased water turbidity due to sand mining-induced pollution and fluctuation in pH levels have been observed in Telangana (Bhatawdekar et al., 2021) and in Kerala along the Chalakudy, Periyar and Muvattupuzha rivers (Sreebha and Padmalal, 2011). The reduction in water quality is further supported by a study conducted by Bhattacharya et al. (2019c) along the Kangsabati River in South Bengal that indicated a very poor pH at Sandchar sites with >70. Water quality and solute fluxes declines have also been reported along many rivers e.g. Manimala in Kerala where the river’s load is estimated to carry 23% of the total dissolved fluxes (Padmalal et al., 2012). These effects have also been observed around the Kadinamkulam estuary (largest estuary in Kerala) with 3.5 km2 of its area especially around Trivandrum affected (Arunkumar and Joseph, 2006) thus translating in a nexus of limited water quality for livelihood and ecosystem use.
In some zones, sand mining has accelerated a reduction in groundwater availability e.g. from the river bed of the Ajay–Mayurakshi of the Ganga River in West Bengal (Sarkar and Islam, 2021). Similar findings of reduced groundwater level and water transparency were observed in Telangana (Bhatawdekar et al., 2021), and in South Bengal due to river channelization (Bhattacharya et al., 2019b). This has also been supported by a study in Kanyakumari where most groundwater aquifers have been polluted or receded (Kaliraj et al., 2015), in Haryana along the Dohan river catchment (Jaglan and Chaudhary, 2014) as well as in the Gauribidanur taluk of Kolar district in Karnataka (Manjunatha et al., 2012) and in Kerala around areas close to the river mouth zones of Lake Vembanad in Kerala e.g. around Muvattupuzha with 116 wet pits (Padmalal et al., 2008).
Most sand mining is conducted using drilling machines to extract high quality sand especially in the lower profiles (Padmalal et al., 2008) and this has led to the creation of riverbank depressions that harbor vectors e.g. in 2020 one mining segment along the Raidak-II River had a depression amounting to 3,838,071 cubic feet (Talukdar and Das, 2021). Other onsite impacts have been reported around the River Aghanashini estuary in Karnataka e.g. excavation impacts and water supply impacts such as channel bed lowering, migration of excavated pits, undermining of structures, bank collapse, caving, bank erosion and valley widening and channel instability (Basavana Gowda et al., 2019).
A paucity of studies have further documented sand mining impacts in coastal zones of India; especially zones with unique black sands (Prasad et al., 2020). Some of the devastating impacts include:
Increased coastal sensitivity to shoreline and morphological changes i.e. erosion and accretion e.g. in Tamil Nadu (Singh et al., 2022), Orissa (Panigrahi, 2005) and along the 41-kilometer stretch of the Paravur-Purakkad coast in Kerala (Prakash Chandar and Prithviraj, 1988); partly due to the drilling of deep holes exceeding 5.5 meters deep e.g. in the East coast of India (Thakur et al., 2014) and around the Tiruchendur zone between Tuticorin and Kanyakumari which have experienced a high linear shoreline change of +530 meters from 1969 to 1999-thus increasing vulnerability to disasters e.g. the 2004 Tsunami (Sheik Mujabar and Chandrasekar, 2013). These have also been reported in the Aghanashini estuary in Karnataka where sand mining has led to the deepening of estuaries along coasts, and the enlargement of river mouths and coastal inlets, leading to saline-water intrusion and rising rates of coastal erosion due to large-scale landscape changes (Basavana Gowda et al., 2019). Another study along the Chavara coast, specifically the Neendakara–Kayamkulam coastal sector in Kerala reported shoreline/coastal caving in e.g. by about 120–200 m at certain critical locations e.g. around the Vellanathuruthu and Kovilthottam mining sites (Prasad et al., 2016). More pronounced shoreline changes have been evidenced around the Tuticorin coast in Tamil Nadu where shoreline retreat rates of –12.04 m/ year and especially around Ovari (retreat exceeding 3 meters/year) (Sheik and Chandrasekar, 2011) due to sand mining-induced erosion and 8.36 m of accretion per year are observed based on Linear Regression Rate (LRR) method. In fact, out of the 100 kilometers’ coastal stretch, 31.7 kilometers especially along Vembar, Periyasamipuram, Kalaignanapuram and Punnakayal villages are more vulnerable with erosion rates of more than –2.0 m/year which has increased risks to sea level rise and coastal floods (Anitha and Usha, 2014) and removal of natural coastal vegetation exposing the coast to strong wave effects e.g. along Navaladi area (Sheik and Chandrasekar, 2011).
Shoreline changes have further mushroomed a reduction in the Dry compressive strength (level of sand grains resistance due to exposure to stresses) of beach sand due to removal (Devi et al., 2023; Pandya et al., 2023) thus increasing beach cave-in e.g. in Tamil Nadu (Elavenil et al., 2017). This has further led to a decline in coastal black sands that naturally protect the coast (reported from about 100% in 1990s to about 24% in 2015 e.g. around Azeekhal along the Chavara coast in Kerala) (Prasad et al., 2016) and a reduction in the supply of nutrient elements and sediments from terrestrial sources e.g. radial rivers from Lake Vembanad around Ernakulam for natural coastal protection (Padmalal et al., 2008). This effect has also been reported around the Tuticorin coast in Tamil Nadu where coastal erosion/accretion rates of –7.9 and 16.3 m/year are reported (Parthasarathy and Natesan, 2015).
In most coastal zones predominated by sand mining, there are increases in saltwater intrusion into coastal aquifers e.g. in the mouth of River Chaliyar in Kerala (Sathya et al., 2023) thus leads to reduced water quality (i.e. short-term turbidity) (Singh et al., 2022). The increased depletion of Quaternary freshwater aquifers has been widely reported around Lake Sasthamkotta in Kerala where; due to the use of high-power jet pumps e.g. at Kadapuzha and along riverbeds e.g. along Kallada river near Kollam, the annual rate of sand extraction of 0.66 million tonnes at 88 sites lowered the water storage zones (Sreekumari et al., 2016). This is supported by a study along the Thamirabarani and Pazhayar rivers in Kanyakumari in Tamil Nadu where the low groundwater aquifers and table level of 0.5 to 5 m has not only been polluted but the entire 34.7 km2 of the coast is highly vulnerable (Kaliraj et al., 2015). Salt water intrusions in estuaries has also been pronounced e.g. around Paravur kayal in Kerala where about 350 truckloads of sand per day are extracted from about 50 sand mining locations/kadavus (Sheeba, 2009).
Another notable impacts has been the increased erosion of beach sediments with several ramifications e.g. affecting groundwater aquifers (Singh et al., 2022). The effect on water aquifers has been accelerated by sand mining-induced seawater pollution. This has also been reported in a study in Pondicherry in Tamil Nadu that increased pollution of seawaters with petro hydrocarbons reaching up to 139 ppm especially due to discharges/oil spills and lithogenic fluxes from sand mining activities (Kamalakannan et al., 2017). A related study in Alappad in Kerala reported that increased coastal erosion from 2005 to 2010 led to a shoreline retreat of 92 m (about 1/5 of the shoreline area) thus affecting fish breeding zones e.g. in Vellanathuruthu and exposure to floods and Tsunami effects (Satish and Geetha, 2021). Another study along the Chavara coast in Kerala reported that increased black sand mining has increased shoreline erosion and inner-shelf morphological changes thus beach cave-in (estimated to have retreated by 300 m from 2000–2022 thus increasing exposure to water pollution, effects of tsunamis and monsoons floods e.g. around Vellanathuruthu–Ponmana and Kovilthottam (Prasad et al., 2020). Related effects were reported in a study around the Tuticorin coast in Tamil Nadu where mining has increased threats of floods, erosion, and sea level rise e.g. rising at the rate of 1.26 mm/year (Parthasarathy and Natesan, 2015).
Furthermore, there is an increase in the destruction of waterway banks and beachfront e.g. in Villupuram in Tamil Nadu where it was found that after every 30 miles of any building site, there is sand mining and this has reduced soil fertility, increased occurrence of natural disasters e.g. soil erosion of beachfront (Ramya and Nivetha Kumari, 2020); due to a decline in the quantity of natural sand that protects the coast (Susheel Kumar et al., 2022). Similar effects of beachfront cave-in were reported in Kayamkulam and Ponmana in Kerala (Prasad et al., 2020) and Tamil Nadu (Elavenil et al., 2017). Along the Ullal-Kotepura beach in Karnataka, there has been the destruction of geo-textile reefs and berms (Jayappa and Deepika, 2018).
More pronounced effects of sand mining (both in river and coastal environs) have been witnessed on extreme biodiversity and ecosystem losses. For instance, sand mining has endangered critical habitats of unique bird species e.g. habitats of critically endangered White-bellied Heron e.g. in Namdapha Tiger Reserve have declined (Menzies et al., 2021). Similar impacts on birdlife communities have been reported in Telangana (Bhatawdekar et al., 2021). Another study conducted in the Cauvery Delta basin in Tamil Nadu from 2017–2019 found that bird habitats e.g. for Asian open bill stork, and the Black-headed ibis have been decimated as sand mining led to negative correlational effects on hydropedological parameters e.g. organic matter and nitrogen and the percent silt content needed by the species (Menon, 2021). These losses have also included habitat loss of avian species habitats e.g. the nesting colonies of the Endangered Black-bellied Tern e.g. along the Godavari River in the Bay of Bengal near Kakinada and about 63 bird species in the Papikonda National Park in Andhra Pradesh (Ray et al., 2020). In Kadalundi Vallikkunnu Community Reserve on the southwest coast of India in Kerala, the habitats (wintering grounds) and nesting grounds around River Periyar in Kerala (Sreebha and Padmalal, 2011) for the migratory shorebirds e.g. on mudflats, mangroves, and sand beaches have been destroyed (Aarif et al., 2020). In fact, a related study by Aarif et al. (2014) reported that shoebird assemblages in the reserve reduced from 67.2% in 2005 to about 37.5% in 2012. Along the Tungabhadra River at Siruguppa, Bellary in Karnataka, there has been a reduced abundance/threat to endangered bird species e.g. for the first time, there has not been any sighting of the Endangered Black-bellied Tern since 2019 (Bayani, 2020). In the Ujjani wetland system in Maharashtra, increased destruction of wetland ecosystems has affected unique bird habitats/communities e.g. of 23% mudflats feeder, 16% upland feeder, 14% marsh feeder, 12% bird of prey, 11% surface feeder and fish eaters and Great Thick-knee that represents one of the Near Threatened category on the International Union for the Conservation of Nature (IUCN) Red List (Karikar et al., 2019) and declines in the endangered gharial have been reported along the Chambal river (Nair et al., 2012).
These effects have also been scaled up in pristine fishing areas where there is increased destruction of fishing zones and habitats e.g. in backwaters, mangroves, estuaries e.g. along the Chavara coast in Kerala where there is a reduction in zooplankton (Sheeba et al., 2004), and inner-shelf morphological changes are affecting breakwater fishing sites e.g. at Kayamkulam; Vellanathuruthu, Ponmana, and Kovilthottam (Prasad et al., 2020), and in the Ujjani wetland zone (Karikar et al., 2019). For instance, in the Ithikkara River, zooplankton for crustaceans, particularly Copepods and rotifers declined from about 78.66% to 33.92% and Meroplanktonic organisms decreased from upstream to downstream regions (Sheeba and Ramanujan, 2005). In the Aghanashini estuary in Karnataka, onsite impacts e.g. increased sedimentation of the river catchment affected the river flow/estuary e.g. annual yield of 1,105 Kilo cum per year observed leading to the destruction of aquatic and riparian habitat, habitat loss, and decreased humus or organic matter (Basavana Gowda et al., 2019). Another study along the Damodar River in between Sillaghat and Sadarghat of West Bengal documented that riverbed ecosystems e.g. fish habitats have been damaged due to use of high-power jet pumps for the extraction of good-quality sand from wet pits and river channel (Ghosh et al., 2016).
In addition, around Lake Sasthamkotta in Kerala, increased sand-induced water pollution has led to a reduction in organic carbon for ecosystems e.g. fish e.g. at Pangod low content of organic carbon (0.02%–0.22%) observed (Sree-kumari et al., 2016). Worse scenarios have also been reported around Neyyar, Peppara and Shendurney sanctuaries and two Reserve Forests of Kulathapuzha, Palode where endemic fish species richness has declined from about 53 to 10 species e.g. in low land areas of undammed rivers (Abraham and Kelkar, 2012). The reductions in fish species have been prominent around river confluences zones e.g. along the Meenachil (Baiju et al., 2009) and especially Ithikkara rivers in Kerala, out of the 56 fish species, 16 species can be enlisted under threatened fresh water fishes e.g. in Kochi and Trivandrum (Sheeba, 2009). In addition, decline of unique edible prey species e.g. Wallago attu and the giant fresh water prawn have been recorded due to a rise in siltation of the feeding grounds affecting detritus and the hatching of eggs e.g. of bivalves on sandy or gravelly substrata (Sheeba, 2009). The impacts on fishes and bird species have further translated into reduced species richness and biota e.g. In a study by Bhattacharya et al. (2019a) along the Kangsabati River in South Bengal an analysis using the Maximum Margalef’s index of species indicated a reduction in species richness in mining sites from 4.62 to 3.64 e.g. around Sandchar sites. In addition, using a Shannon–Wiener diversity index, results indicated a reduction in biota in mining sites of 0.11, 0.89, 9.23, and −1.39 and pit sites 0.12, 0.90, 10.68, and −1.50 in three sampled mining zones. In the Vann Island region in Tamil Nadu, sand mining has further reduced water quality in the Thambrabarani estuary affecting marine biota (Jayaprakash et al., 2016). In the Cauvery river basin of Karnataka and Tamil Nadu, the abundance of riparian ecosystem species e.g. smooth-coated otter abundance reduced from about 38% to 7% (Sunil et al., 2010).
In other words, sand mining activities have caused immense damages to the biodiversity of the riverine and marine ecosystem e.g. Chaliyar River in Kerala (Sathya et al., 2023) e.g. a study along R. Chambal in Northern India reported mass destruction of the aquatic life population (Singh et al., 2022). Another study in the River Ganga region found that sand mining has led to a decline in 29 macro-benthic species number in 4 zones of the river caused by habitat destruction in the form of increasing depth, slope, channelization of river, and water quality (Kamboj and Kamboj, 2021). In the Marine Protected Area around the Gulf of Mannar in Tamil Nadu, habitats e.g. of endemic and endangered species, including Dugong and resources e.g. mangroves, mudflats e.g. around Korampallam Odai and Tha-miraparani estuary are increasingly threatened (Parthasarathy and Natesan, 2015). These damages have also affected small rivers e.g. in South western India especially in Kerala (Padmalal and Maya, 2014a). In addition, the biochemical composition and total biomass in beach ecosystems have been under threat e.g. sedimentary microbes other than bacteria e.g. the protozoans e.g. species population declined from 1.99 to 0.45 × 106 cells g−1 sediment around Kalbadevi beach, in Ratnagiri, in the West Coast of India (Fernandes et al., 2012). Along the Nila river in Kerala, benthic fauna e.g. of nematodes, crustaceans, insects and mollusks. Reduced e.g. a survey of section IV of the river indicated a decline of 11.59% (Sushama and Radhakrishnan, 2010).
These impacts are further evident with the scale of ecological damage in ecologically sensitive zones e.g. to coastal estuaries (Bhatawdekar et al., 2022) thus leading to the depletion of some natural resources (Prakash Chandar and Loganathan, 2022). Another study by Bisht (2019) revealed that the destruction of ecological sites has been observed e.g. in the Gwalior district of Madhya Pradesh where the critically endangered amphibian-the gharial in the National Chambal Wildlife Sanctuary is threatened. In Tamil Nadu around the Gulf of Mannar, there has been the destruction of the soft coral zones around Vann fringing reef island due to increased pollution e.g. around the Thambrabarani estuary. In addition, there is increasing evidence of the shrinking of Vann fringing reef island e.g. from 265,479 m2 in 1973 to 40,733 m2 in 2016 as sand mining has partly contributed to the weakening of sediments (Jayaprakash et al., 2016).
Other observed impacts have related to Microclimate changes and formation of heat islands within the urban area, e.g. temperature increase (average maximum temperature increased by 2°C from 2001–2013) e.g. along the Cauvery and Coleroon River and around Srirangam Island, Tiruchirappalli district of Tamil Nadu due to removal of vegetation (Muthamilselvan et al., 2016). This partly indicates that the overall impacts of sand mining could scale-up climate change effects as it not only affects the environmental ecosystem, but also opens up new climate-related challenges which either lead to increased temperature or a reduction in precipitation.
6. Implications on Livelihoods
The impacts of sand mining on the environment have directly translated into the decimation of livelihoods that highly depend on nature; especially among vulnerable communities (Menon, 2021). These impacts are shown in (Table 3) and they include:
Disruption of agricultural activities e.g. paddy rice farming e.g. along river Chambal in Northern India due to increased loss of groundwater (Singh et al., 2022), flooding e.g. along the Rarh plain of the Bengal basin (Islam et al., 2022), Effect on fruit and vegetable production due to subsidence and recession of riverbank e.g. in the Dooars region of West Bengal along Raidak-II River (Talukdar and Das, 2021). Destruction of Paddy fields has affected a broad range of habitats to wintering waterbirds as natural wetlands e.g. in the Cauvery delta basin, in Tamil Nadu (Menon, 2021). Similar findings were reported in a study by Jana (2021) along the Subarnarekha River where there has been an acute loss of riparian arable land and crop damage. In addition, there has been a loss of fertile cropland along meandering alluvial banks e.g. floodplain of the Bhagirathi-Hooghly river delta in West Bengal is dying (Ghosh et al., 2020). A study along River Kangsabati in South Bengal categorically quantifies the extent of loss of fertile soils in the flood plains due to sand mining. It is reported that the maximum bank erosion of fertile soils in the Mohanpur 3 section was 79.3 meters and at Kapastikri 1 section, it reached 50.02 m per year (Bhattacharya et al., 2019b) and in the Aghanashini estuary in Karnataka, floodplain alluvium reduced between 61.5 to 71.5 cm per month between 2014–2015 (Ramachandra et al., 2018).
Another study around Lake Sasthamkotta (the largest in SW India) in Kerala astoundingly reported that the pit lakes and deep pools left after floodplain mining increased from 2.5 km2 in 1999 to 3.57 km2 by 2014 affecting paddy rice farming (Sreekumari et al., 2016). Similar findings have been reported along the Palaspai lower course of River Kangsabati in West Bengal as sand excavation has accelerated river rejuvenation, especially in the post-monsoon periods (Bha-ttacharya et al., 2016). Related effects in the floodplain have been reported in Hanuman Nathi and Uppar in Tamil Nadu where there was a net decrease in alluvial sediments of 0.778 km2 with a rate of 25,933 m2/year (Sheik Mujabar and Chandrasekar, 2013). In some cases, sand mining activities by large mining companies have wiped out entire farmlands of marginalized people e.g. in Muriyad in Kerala (Bisht and Gerber, 2017). In Kanyakumari in Tamil Nadu, 42% of farmlands are either polluted or sand mining-induced deep pools have been created (Kaliraj et al., 2015). A study among 90 farmers in Gauribidanur taluk of Kolar district in Karnataka by Manjunatha et al. (2012) puts these impacts into perspective as it reported that pollution of irrigation streams and soils has impacted the crop planting patterns and kharif seasons on dry lands on to which 45,194 farmers and 7,256 livestock depended. In addition, the gross cropped area under sugarcane (25 percent), paddy (2.4 percent), ragi (1.2 percent), and areca nut (1.2 percent) was reduced to zero leading to a loss of 2,043 rupees per acre. Increased costs of drilling groundwater irrigation well for cropping have also been observed due to the lowering of the water table. In addition, 100 percent of livestock farmers reported an increase in expenditure on livestock health of about 4,112 rupees per year either due to pollution-induced complications or lack of water sources. The gross impacts on agriculture have been ballooned by the reduction of water for irrigation e.g. western and eastern banks of the Bhagirathi–Hooghly River (Sarkar and Islam, 2021), also reported in the Lower Damodar River due to flow diversion and poor water quality (Bhattacharya and Das Chatterjee, 2021) and sedimentation of reservoirs for hydroelectricity e.g. around Achankovil, Pamba, and Manimala in Kerala (Padmalal et al., 2008).
In most fishing zones, sand mining has disrupted wild fishing activities e.g. negative impacts observed on crustacean and mollusk populations and fish breeding sites e.g. in Pakke Tiger Reserve in West Bengal due to the use of destructive techniques e.g. explosives (Menzies et al., 2021); Destruction of riverbanks small fish breeding/fishing sites e.g. in the Dooars region of West Bengal along Raidak-II River (Talukdar and Das, 2021), similar findings reported in downstream fishing regions in Telangana (Bhatawdekar et al., 2021), Reduced backwater fish availability e.g. in Vellanathuruthu in Alappad in Kerala (Satish and Geetha, 2021). A related study in Kadalundi Vallikkunnu Community Reserve in Kerala reported increased destruction of estuaries and mangrove ecosystems-a habitat for clams (Aarif et al., 2020). These findings are consistent with a study carried out around Lake Vembanad in Kerala (Sebastian et al., 2012). The increased loss of fisheries livelihood and fishing zones (Jayappa and Deepika, 2018) have been reported around the Aghanashini estuary in Karnataka where bivalve and fish catch declined by about 40%–50% from 2015–2018 (Ra-machandra et al., 2018) and in three wildlife sanctuaries of Neyyar, Peppara and Shendurney and two Reserve Forests of Kulathapuzha, Palode in Kerala mainly due to sand mining induced pollution of fish dwelling substrates (Abraham and Kelkar, 2012). In coral reef zones of Tamil Nadu, there is an increase in the destruction of rich reef fishing zones and fishery resources e.g. Around the Thambrabarani estuary (Jayaprakash et al., 2016). The devastating effects of coastal sand mining have been reported in a study along a 100 km stretch in Tuticorin in Tamil Nadu where 25 fishing villages covering 31.7 km e.g. Vembar, Periyasamipuram, Kalaignanapuram, and Punnakayal have their livelihoods ranked under very high-risk category (Anitha and Usha, 2014).
Similar effects along the Tuticorin coast have been reported in a study by (Sheik Mujabar and Chandrasekar, 2013) that the shoreline retreat of 530 m from 1969–1999 has decimated estuarine fishing. These impacts have led to the overall loss and reduction in fisheries wealth e.g. of the unique traditional fishes e.g. the edible Wallago attu fish e.g. along the Ithikkara river in Kerala. Survey research among fishermen found that over 75% of respondents reported no catch of the species in the last 5–6 years (Sheeba, 2009). These impacts have further been evident among communities engaged in mariculture and aquaculture where there has been the destruction of aquaculture and fish farms e.g. in the Rarh Plain of the Bengal basin due to increased flooding (Islam et al., 2022). Additional threats to aquaculture farms have been reported e.g. in the estuaries e.g. around Koram-pallam Odai and Thamiraparani estuary, along Tuticorin and Gulf of Mannar in Tamil Nadu where sand mining erosion/accretion rates of –7.9 and 16.3 m/year are reported (Parthasarathy and Natesan, 2015). These impacts have also been observed along the Chalakudy, Periyar, and Muvattupuzha rivers, in Kerala e.g. near Kochi where the 96 active mining pits in the Muvattupuzha floodplain have polluted fish farms due to oil/ gasoline spillage (Sreebha and Padmalal, 2011).
Most sand mining is conducted in areas with sedentary communities and this has increased Conflicts on the use of natural resources (Prakash Chandar and Loganathan, 2022), e.g. with small fisher communities, and paddy farmers in Udupi, Karnataka (Szabo and Hegde, 2021). This has led to the displacement of coastal people e.g. in Vellanathuruthu in Alappad (Satish and Geetha, 2021). Similar findings on settlement loss due to displacement are reported along the Bhagirathi-Hooghly river delta in West Bengal (Ghosh et al., 2020). A study by Bisht (2019) documented that 45% were related to illegal extraction of sand, discontentment, and resistance against large-scale sand mining activities and these have led to gross life consequences e.g. led to the death of activist-Swami Nigamananda Saraswati in 2011 after a 4-month fasting protest and sand mining-related deaths are currently estimated to be over hundreds annually in India. The escalation in conflicts has been observed in most of India’s sand mining states e.g. Gujarat. For instance, a study of sand mining cases from 1994 to 2014 revealed that Tamil Nadu, Odisha, Kerala, and Karnataka experienced the highest frequency of local conflicts and resistance against unfair ecological distribution rights (EDCs) meted out to vulnerable communities e.g. among Muriyad communities in Kerala against larger mining corporations. In fact, 75% of social activists movements against EDCs in sand mining zones are led by either marginalized, rural, or indigenous communities e.g. the Adivasi (Bisht and Gerber, 2017). In addition, environmental and social injustice against marginalized communities has been proliferated e.g. in Udupi in Karnataka (Szabo and Hegde, 2021). In some areas, there is loss of land access as land is leased to sand mining dealers e.g. in the Dooars region of West Bengal along Raidak-II River (Talukdar and Das, 2021). Other examples related to loss of land are reported by coastal people e.g. in Vellanathuruthu in Alappad (Satish and Geetha, 2021), and along Subarnarekha river, where local communities have lost land rights (Jana, 2021). Similar social injustices meted out to vulnerable communities on the use of poromboke-commons land e.g. in Tamil Nadu have been reportedly increasing (Bisht, 2019).
The loss of resource access rights has reduced the provision/access of a source of fresh drinking water e.g. to over 700,000 people around Lake Sasthamkotta (the largest in SW India) in Kerala (Sreekumari et al., 2016). The devastating impacts have been reported in Gauribidanur taluk of Kolar district in Karnataka where deduced clean surface water availability for drinking has increased the daily transaction cost for drinking water to 30 rupees and an additional expenditure of 1,848 rupees per family towards fetching potable water (Manjunatha et al., 2012). The increasing scarcity of clean drinking water has further been reported around the Manimala River in Kerala in Scarcity of drinking water e.g. the river has become more acidic throughout the year with pH ranges of 6.29–6.94 during non-monsoon and 5.9–6.5 during Monsoon periods e.g. at Mundakkayam, Manimala, and Thondara (Padmalal et al., 2012). Similar effects have been reported around Kochi e.g. along River Manimala where over 60% of the wells in its lower profile experience acute safe water shortage problems (Sreebha and Padmalal, 2011).
Sand mining has also led to critical health concerns as miners and communities near mining sites are exposed to toxic mineral compounds e.g. e UV–VIS-NIR. For instance studies of ilmenite e.g. in Chavara in Kerala and Manavalakurichi in Tamil Nadu mainly show two peaks around 350 to 500 nm and 1,300 to 1,370 nm (Sundararajan et al., 2021). In some heavy placer mining zones (Prasad et al., 2020), there has been an increase in reports on chromosome and chromatid damages e.g. DNA Aberrations including gaps, breaks, acentric fragments, exchanges, dicentrics, and polyploids changes among miners due to exposure to Naturally Occurring Radioactive Materials (NORM) from placer minerals. Other related health risks in mining zones e.g. reported in Haryana include increased cardiovascular complications due to smoking, alcoholism, and occupational exposure to genotoxic compounds (e.g. heavy metals, silica, etc.) and also due to the low doses of ionizing radiation from NORM present in the beach sand (Meenakshi and Mohankumar, 2013). Another study in Jaduguda and Singhbhum Thrust Belt in Bihar supports this finding as it found a significant correlation between sand mining and increased exposure by miners to airborne respirable ore dust and the associated a-rays of long-lived radionuclides concentrations in the mine leading to conventional inhalation hazards and health risks (Jha et al., 2000). Most studies on heavy placer mining in India confirm that exposure of miners’ increases chances of acute health defects. For instance, a study by Paul et al. (1994) reported a significant relationship between toxic radiation exposure and monazite extraction in coastal sands e.g. in Kerala (Prasad et al., 2020). e.g. In Kerala for instance, monazite levels on most houses had higher levels ranging from 220 Rn to over 420 Bq m[sup -3]. In the urine samples of adult miners e.g. in Manavalakurichi, toxic compounds from radiation were also high ranging from 232 Th and 228 Ra (Paul et al., 1994).
Sand mining has also affected tourist/sacred cultural-religious and recreation picnic sites e.g. due to changes in river bank morphology (Menzies et al., 2021). This has also been a result of the destruction of floodplain rice fields that are a habitat for wintering waterbirds e.g. the Black-headed ibis in the natural wetlands e.g. in the Cauvery delta basin, in Tamil Nadu (Menon, 2021). A related study in Papikonda National Park in Andhra Pradesh from 2017–2018 reported Negative effects on bird-watching tourism e.g. along the Godavari River in the Bay of Bengal near Kakinada-reduced sightings, especially in December and August (Ray et al., 2020). Similar threats to bird tourism have been reported in Maharashtra, especially in the Ujjani wetland system where unique species e.g. the Great Thick Knee are now categorized under the IUCN Red List of endangered birds (Karikar et al., 2019). In other words, the proliferation of sand mining is affecting conservation tourism in historical reserves e.g. The Kadalundi-Vallikkunnu Community Reserve in Kerala (Aarif et al., 2014). Coastal beach erosion affects tourism e.g. around Kollam and Azeekhal in Kerala (Prasad et al., 2020). A similar finding was reported along Ullal-Kotepura beach in Karnataka where recreational beachfronts were destroyed (Jayappa and Deepika, 2018). Destruction of sacred religious and cultural sites along rivers e.g. along the Ganga (Bisht, 2019). Around Vann Island in Tamil Nadu, the destruction of soft coral reef zones has decimated coral reef tourism revenue (Jayaprakash et al., 2016). In addition, along Chandernagore in Hooghly along the Ganges Delta, riverbank structures e.g. religious temple and cultural sites e.g. where Puja (a form of Goddess “Durga” seated on the lion)-dating back earlier than 1750 has collapsed and been destroyed (Saha, 2015) affecting aesthetics. The damages to cultural and religious temples have also been reported around Achankovil, Pamba, and Manimala in Kerala (Padmalal et al., 2008).
Destruction of infrastructures e.g. bridges and hydraulic structures e.g. in Kerala (Sathya et al., 2023). A related study in Kerala along the Chavara coast further reported increased destruction/weakening of coastal defense structures like breakwaters, groins, and seawalls e.g. around Srayikkadu and Ponmana (Prasad et al., 2020), along the Neendakara–Kayamkulam coastal sector in Kerala (Prasad et al., 2016) and in Karnataka e.g. along Ullal-Kotepura beach (Jayappa and Deepika, 2018). Similar offsite impacts especially on transport infrastructure have been reported around the Aghanashini estuary in Karnataka where livelihood infra-structure e.g. railway bridges at Kaiyari and Dundukuli have collapsed due to sand mining deep pools and morphological changes (Ramachandra et al., 2018). Around the Damodar River in between Sillaghat and Sadarghat of West Bengal, there has been an increase in the collapse of houses for settlement (Ghosh et al., 2016). Other infrastructural damages have occurred on engineering structures like bridges, water intake structures, side protection walls, and spillways around Lake Vembanad in Kerala (Padmalal et al., 2008). These findings are supported by research by Singh and Dhar (1997) in the southern coastal belt of India that reported that coastal mining has led to the emergence of sinkholes that destroy and beak coastal infrastructure. The destruction of infrastructure and competition for sand has increased the cost of construction materials (sand becoming expensive) (Devi et al., 2023).
One of the worst effects of sand mining has been the emergence and increasing influence of sand mafias e.g. in Telangana and in some reports, almost 50% of the 10 million cubic meters of sand per year comes from illegally exploited coastal sands by mafia dealers (Bhatawdekar et al., 2021). A study in Villupuram, Tamil Nadu reported that sand mafias connections and influence e.g. led to the loss of government jobs e.g. by an activist-Durga Shakti Nagpal, and threats to local people opposing sand extraction (Ramya and Nivetha Kumari, 2020). In addition, the increased emergence of mafias and powerful cartels e.g. in Tuticorin that extract in common-user lands (from the permitted 4 hectares of leased land to over more than 30 hectares of poromboke [commons] land has led to an increase in illegal sand mining trade e.g. in Vaipar, Vembar, and Periyasamipuram beaches in Tamil Nadu, where over 230,000 tonnes of beach sand are illegally mined and traded. This does not only lead to loss of government revenue, but is also a legal offense liable to punishment under charges of theft under the indian penal code, Coastal Regulation Zone notification, 2006, and under various Acts of the States e.g. the Tamil Nadu Public Properties Prevention of Damage and Loss Act, 1992, Illicit Mining and Minerals Act, Environmental Protection Act, 1986 (Bisht, 2019). The mafia connections have further led to increased transboundary illegal sand mining trade e.g. between India and Malaysia (Susheel Kumar et al., 2022) between India and dealers in the Southeast Asia (Bhatawdekar et al., 2021) e.g. in Vietnam, Cambodia, and Indonesia-have become suppliers of aggregate materials-through legal or illegal sources (supported by India River’s Forum Report 2020, Mahadevan, 2019; Marschke and Rousseau, 2022). This partly implies that sand mining effects are increasingly becoming transnational as the demand for sand rises (IRP, 2020) and this could have several ramifications in the global governance systems.
7. Implications for Sustainable Development and Governance
The reviewed documents clearly show that sand mining effects significantly represent a new paradox that directly derails the attainment of SDGs and livelihood targets (Susheel Kumar et al., 2022). In addition, in some studies e.g. by Bhatawdekar et al. (2021) it is indicated that there is a sand governance challenge as most dealers either directly mine in protected zones under the Coastal Zone Management regime of India or hardly pay taxes or threaten local communities limiting access to livelihood resources e.g. water (Sreekumari et al., 2016). In addition, the increase in illegal transnational sand trade e.g. as reported between India and other Asian countries e.g. Malyasia (Susheel Kumar et al., 2022); Cambodia and Vietnam (Bhatawdekar et al., 2021) directly imply that the scale of damage is no longer localized but becoming complex regional and global phenomena (Mahadevan, 2019). This could partly explain for the increase in social-environmental injustices and rise of social activism and violence as reported by (Bisht and Gerber, 2017) to reap from the lucrative sand mining trade (India River’s Forum, 2020).
Worse still, if we conceptualize the dilemma associated with sand mining on livelihoods and environment, it is easy to contend that sustainable development targets in India’s case are increasingly being threatened as Sand mining effects are directly meted onto livelihood capitals and environmental resources (Marschke and Rousseau, 2022) that are a benchmark to gauge the level of sustainable development (World Ocean Assessment II, 2023; UN-SDGs Report, 2023). For instance, environmental impacts (Table 2) e.g. on ecosystems and their diversity (Sathya et al., 2023), on rivers (Sathya et al., 2023) and coastal zones (Singh et al., 2022) directly affect SDGs 14 and 15. In addition, as reported by Muthamilselvan et al. (2016), there is an increasing correlational threat to SDG 13 on climate action especially in coastal urban zones.
Sand mining implications on sustainable development in the context of sustainable livelihoods are well illustrated in Table 3. For instance, effects on agriculture (Singh et al., 2022) and fisheries (Islam et al., 2022) have gross ramifications on SDGs 1, 2, and 12. In addition, the restriction of access to resources (Prakash Chandar and Loganathan, 2022) and vulnerable people’s marginalization (Bisht, 2019) not only accelerates social and environmental injustices (Szabo and Hegde, 2021) but derails the attainment of SDGs 10, 16, and 11. The effects on infrastructure (Devi et al., 2023; Sathya et al., 2023) have direct ramifications on SDG 9. Water-related issues and challenges (Sreekumari et al., 2016) imply lackluster progress toward SDG 6 and health issues due to mining (Ghosh et al., 2016; Sundararajan et al., 2021) demonstrate challenges to SDGs 8 and 3. In other words, the sand mining effect has a direct impact (mainly negative) on livelihood and the environment and this could frustrate India’s agenda for sustainable and inclusive development.
8. Implications of Sand Mining in the Context of the Different Sand Mining Zones/Regions e.g. in the Global South
In other words, the findings obtained from the review clearly documents that the effects of sand mining cascade through local contexts and are transboundary e.g. in the case of India where for instance, riverbank sand mining has scaled up long-term effects e.g. on biodiversity in areas far away from the source/extraction zones (Mahadevan, 2019). This perspective on the localized complexities emanating from sand mining that are being felt in threshold zones are clearly explained in a study by Matovu et al. (2024) on the resource nexus perspectives in the context of Kerala. This partly means that regions that experience localized increases in sand mining are likely to increase multiplier externalities to threshold areas far away from source regions (Fletcher et al., 2021). For instance, as reported in some states e.g. Kerala, it is observed that sand mining in places e.g. Kollam has created multiplier effects along rivers e.g. Chaliyar extending to over 100 km (Matovu et al., 2024; Sreekumari et al., 2016).
In the context of other countries in the Global South, several reports concur that sand mining is increasingly becoming the new wicked challenge to sustainability; partly due to less regulatory mechanisms and the covert nature of the effects (Jouffray et al., 2023) and this is already jeopardizing ocean sustainability agenda. For instance the 2022 Ocean Risk and Resilience Action Alliance (ORRAA) Report clearly puts this into persctive as it reported that countries in Africa e.g. Senegal, Uganda and Mozambique are experiencing unprecedented environmental shocks e.g. flash floods in sand mining extraction zones e.g. flash floods that swept away Katonga bridge near lake Victoria; partly attributed to illicit sand mining extraction in Lwera swamps. This could partly imply that local communities and resources sedentary near sand mining zones are likely to experience new waves of environmental risks (Matovu et al., 2024).
In parts of South Asia, the effects have been reported to be multi-dimensional (Mahadevan, 2019). This is clearly reported in studies e.g. by Marschke and Rousseau, 2022 that found out that the construction boom in India could be a catalyst for regional sand mining increases as sand mining dealers e.g. in Cambodia, and Thailand seek to trade in more sand. This observation is clearly demonstrated in a study on sand mining in Kerala that reported increased international sand mining inflows to Indian states from other Asian nations (IRP, 2020; Matovu et al., 2024).
More complex challenges in different case studies e.g. in Latin America e.g. Chile and in Africa e.g. Senegal are reported in studies by Arboleda (2020); Engels and Dietz (2017); and Vesalon and Creţan and Vesalon (2017) that unmistakably offer a fresh worldwide viewpoint on the conflicts that mineral extractivism e.g. of sand may give rise to locally or globally. Arboleda (2020), for example, noted that growing capital investments in the sector are generating new transboundary global “planetary conflicts and governance complexities,” particularly in developing regions of Asia, Africa, and Latin America. This is contributing to an increase in imperialist practices, such as the violent eviction of people from coastal lands that support their livelihoods and the overtly capitalistic nature of increased extraction i.e. sand mining; if not regulated might bubble into secondary livelihood challenges e.g. related to land evictions (Matovu et al., 2024).
In addition, further evidence on sand mining complexities is provided in Engels and Dietz’s (2017) book, which shows that growing urbanization e.g. in developing countries e.g. Ethiopia has led to a boom in extractivist resources demand both spatially and temporally. This has negatively impacted local communities by causing a web of localized conflicts, institutional and structural barriers to resource trade regulation, and actor constellations. Creţon and Vesalon (2017) also reported on this evidence e.g. in Latin America and Europe regarding the intersectionality between sand mining, ecosystems, and livelihoods. They found that the root cause of the complex institutional settings and political and economic systems that currently exist in most regions of the global South, as well as the increased mining of resources like gold, oil, and currently coastal and riverine sand, is increased mining. To put it simply, sand mining activities are likely to jeopardize sustainability concerns and may have more impact on the sustainability of resources and ecosystems in the future (Matovu et al., 2024). A comparative case study research by Jouffrey et al. (2023) clearly compounds the negative viewpoints on how a new network of covert local sand mining actors connected to both political and economic institutions is being used by imperialist entities to pounce on less controlled resource zones in developing countries.
9. Conclusion
Our study clearly presents a case for an increasing focus on the complexity of sand mining not only in India but also in most source jurisdictions. Based on the gross negative implications of sand mining across the livelihood and environmental domains, we can augment that sand mining is an invisible wicked challenge that could derail the attainment of sustainable development at both micro and macro levels. In addition, we observe a cobweb of system interactions in the sand mining chain that even threatens local and regional governance. Since there is a reported lack of strong mechanisms to mitigate the negative externalities, we suggest the development of integrated sand mining management approaches and legal mechanisms from micro and macro levels. This could be through local governance actions/initiatives e.g. the mapping of allowed and non-allowed sand mining zones; especially along river channels and marine zones, use of integrated local stakeholder governance practices e.g. local sand governance and management action plans, and at a macro level, initiatives could involve increasing research in the use of alternative material e.g. sawdust (Devi et al., 2023), marble slurry dust instead of crusher sand (Murugesan et al., 2020), laterite quarry waste (unprocessed laterite), and with the processed laterite (Basavana Gowda et al., 2019), waste foundry sand in concrete as a substitute for fine aggregate (Nithya et al., 2017), M-sand manufactured from Garnetiferous Biotite Gneiss (GBG) and belonging to Zone II grade (Anand and Reddy, 2014), that can replace or minimize or partially substitute the overuse of sand and designing of a new sand mining and transnational trade governance mechanism e.g. certification mechanisms to monitor the sand mining value chains e.g. marking and branding of sand. The urgent need for a new paradigm and governance shift is clearly documented in the 2022 ORRAA report that calls for urgent research and development of governance mechanisms to regulate sand mining e.g. in developing countries. A study by Jouffrey et al. (2023) further clearly puts this into perspective as it warned that unless new mechanisms are established, their perspective may be harmful to the viability of sustainability in the future and a forerunner to social conflict, environmental upheavals, uneven development, and further marginalization of communities.