1.Introduction
Korean tidal flats are highly productive due to the important food source that they contain, which is a combination of mineral-rich sediments and microalgal organisms, also known as microphytobenthos (Koh et al., 2006). Recent studies show that daily productivity of the tidal flats in Gyeonggi Bay reached ~1,000 mg C m−2 d−1 (Kwon et al., 2014). Thanks to this high productivity, Korean tidal flats produce annually about 50,000–90,000 tons of clams, over 1,000 tons of mud octopuses, and 500 tons of polychaetes (Je et al., 2013).
Given that tidal flats are economically important in Korea, it is essential to know the economic value of the tidal flats for establishing conservation policies. It is especially important to know the monetary value of the items that can be traded in the market and receive market values. Since the economic value of tidal flat fishery can be relatively easily identified, this paper will focus on identifying the value of the carbon stored in these tidal flats. Although there are a number of studies on the monetary value of blue carbon, little research has been done about the carbon value of Korean tidal flats.
The assessment of the value of carbon stored in the tidal flats can be used as an incentive for coastal conservation, such as Marine Protected Area (MPA) establishment and management, coastal wetlands restoration, and sustainable fisheries. The awareness of blue carbon value can also help to prevent development that fails to consider coastal ecosystem service values, which form the opportunity cost. In addition, this value recognition can function as a stimulus for the restoration of coastal wetlands through voluntary carbon offset markets or related carbon valuations in Korea. Payments for ecosystem services, whether part of offset markets or not, also require a calculation of any blue carbon’s economic value to set the appropriate valuation or payment (such as the minimum willingness to accept). This report therefore examines the amount and value of carbon stored in the tidal flats in Korea and recommends ways in which to use the carbon storage value for tidal flat conservation policies.
2. Tidal Flats for Carbon Repository
Carbon sequestered in vegetated coastal ecosystems, specifically mangrove forests, seagrass beds, and salt marshes, has been termed “blue carbon” (McLeod et al., 2011). McLeod et al. (2011) explain that this is because vegetated coastal areas can sequester more carbon from both internal and external sources than unvegetated coastal areas. However, some studies show that even unvegetated tidal flats can sequester large amounts of carbon in areas with high levels of mud (Chmura et al., 2003; Sanders et al., 2010). It is not surprising that mudflats have a rapid and high carbon sequestration capacity. This is because mudflats have a lower permeability, so they bind depositional organic matter for a long time, and also have a high primary yield due to a large number of microorganisms such as cyanobacteria.
Recently, at the 13th Meeting of the Conference of the Contracting Parties to the Ramsar Convention on Wetlands, Parties adopted the “Resolution XIII.14: Promoting conservation, restoration and sustainable management of coastal blue-carbon ecosystems.” In this Resolution, blue carbon is defined as “The carbon captured by living organisms in coastal (e.g. mangroves, saltmarshes and seagrasses) and marine ecosystems and stored in biomass and sediments” with the following contents:
“4. NOTING that the United Nations General Assembly (UNGA), in Resolution A/RES/71/257 on Oceans and the law of the sea, notes the vital role that coastal blue-carbon ecosystems, including mangroves, tidal marshes, and seagrasses3, play in climate adaptation and mitigation through carbon sequestration, […]”
“3 Unvegetated mudflats and intertidal marshes are also important blue-carbon ecosystems. […]”
It is worth noting that, according to the Resolution, unvegetated mudflats are now being recognized as an important blue carbon repository. The International Union for Conservation of Nature has also highlighted their importance, acting as more efficient carbon pools than tropical rainforests (IUCN, 2017). They have the potential to contribute significantly to the achievement of the United Nation’s Sustainable Development Goal 13 on climate action, as a measure that can be integrated into national policies (target 13.2) (UN, online). Specifically, UNEP’s Blue Carbon Initiative has recently highlighted the contribution of coastal wetlands, including tidal saltmarshes, to national targets of carbon reduction (Thomas et al., 2020). This can also assist in the wider achievement of reversing the degradation of marine and coastal habitats and maintaining the amount of carbon sequestration.
Korea’s blue carbon research is in its early stages. A national-level survey was launched in 2018, conducted by Seoul National University (SNU), and funded by the Ministry of Oceans and Fisheries (MOF) and Korea Marine Environment Management (KOEM). This assessment aimed to identify the total amount of organic carbon present in the form of organic matter in the tidal flat. It concluded that the accumulation of organic carbon in the tidal flats varies depending on the vegetation and soil characteristics of the survey area (Table 1). The mean organic carbon of total surveyed areas is 57.58 t C ha−1. When comparing soil characteristics, tidal mudflats have an average of 2.3 times more carbon than tidal sandflats.
The area of tidal flats in Korea was calculated as 250,000 ha in 2013. To estimate the total amount of carbon stored in the tidal flats, this area was multiplied by the mean value of the 2018 survey, 57.58 t C ha–1, resulting in 14.4×106 t C. Assuming that all carbon stored in the tidal flats is released into the atmosphere, the potential CO2 emissions from the tidal flats is 52.85×106 t CO2 e (carbon dioxide equivalent); this is calculated by multiplying C stocks by 3.67, the molecular weight ratio of CO2 to C (Table 2). Given that Korea’s total amount of greenhouse gas (GHG) emission in 2016 was reported as 694.1×106 t CO2 e (MOE, 2018), the potential tidal flats emission corresponds to around 8% of total GHG emissions.
The direct effect of reclamation is that organic matter in the soil is oxidized, and carbon dioxide is released into the atmosphere as the tidal flat turns into land. Thus, former tidal flats, where reclamation has been carried out, are losing their historically-stored carbon (Philip Williams & Associates and Science Applications International, 2009).
According to the recent study of Kwon and Khim (2018), carbon stocks in reclaimed areas have dramatically reduced, and now average 0.27 t C ha−1. Considering the mean carbon stock in the Korean tidal flats is 57.58 t C ha−1, the net decrease by reclamation is calculated to be 57.31 t C ha−1. Given that around 170,000 ha have been reclaimed since the late 1970s (Koh and Khim, 2014), the amount of sequestrated blue carbon has decreased around 9.74×106 t C. The CO2 emissions from previous reclamation is calculated to be 35.8×106 t CO2 e.
3. Monetary Value of Carbon Stored in Tidal Flats
To evaluate how blue carbon can serve as an economic incentive to protect essential coastal habitats from development, this report aims to calculate the monetary value of blue carbon. For more specific data, Ganghwa tidal flat (Figure 1) was selected because of its location, use and conservation state. This area consists of muddy soil, and Table 3 shows the mean organic carbon stock (t C ha−1) and the carbon dioxide equivalent (t CO2 e ha−1) that may potentially be emitted in the form of carbon dioxide.
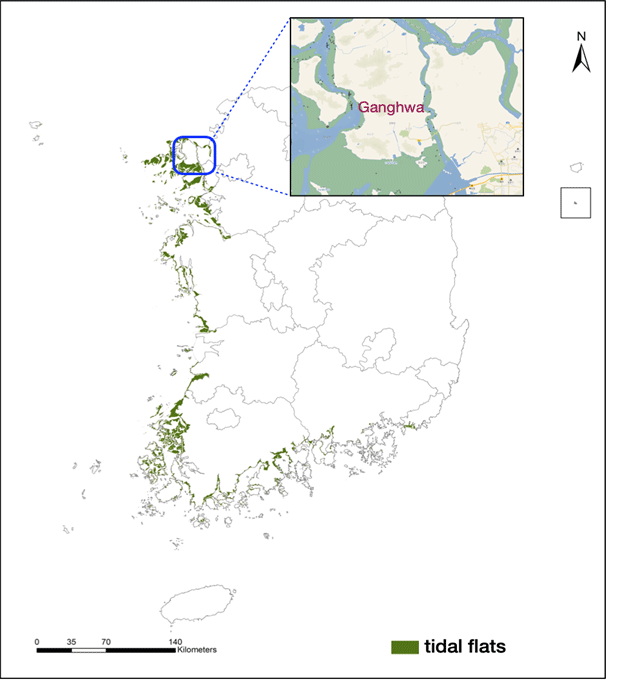
Location | Mean organic carbona(t C ha−1) | CO2 equivalent (t CO2 e ha−1) | Remarks |
---|---|---|---|
Ganghwa | 95.15 | 349.20 | Incheon Metropolitan City, |
The mouth of Han River, | |||
Presence of high development pressure. |
To evaluate the present value (PV) of carbon stocks as a public good, it is important to use the appropriate discount rate and time periods, since these factors yield very different outcomes. The Korean government recently reduced the social discount rate from 5.5% to 4.5%, by revising the ‘Guideline for Conducting Preliminary Feasibility Studies’ in 2017. This social discount rate is being used in analyzing the economic feasibility of public investment projects (NLIC, 2019). Accordingly, it is reasonable to apply a 4.5% discount rate, which is the ‘administrative rule.’ However, considering the sensitivity of PV to the discount rate, this paper also examines the case of applying a lower rate (2%) and a higher rate (7%).
Lopez (2008) noted that the appropriate discount rate should depend on the horizon of the project; he applied 25-year horizons for a 4.4% discount rate in his study. In another study for blue carbon payment, Murray et al. (2011) used a 25-year time horizon based on a decay function of biomass and organic matters in soil. This is because most organic carbon in biomass and soil is emitted to the atmosphere during the 25 years after conversion and disturbance. Considering this carbon longevity in soil and the Korean government’s official discount rate of 4.5%, it is compelling to use a 25-year time horizon.
Using the estimation of sequestration rate and carbon stock in the Ganghwa tidal flat (Table 4), this paper evaluates the monetary value of blue carbon benefits. Only the top meter of soil pool is used for the estimation of carbon storage and monetary value, because most of the vulnerable organic carbon is stored within a meter of the soil’s surface. Since the annual carbon burial rate of Korea’s tidal flats has not been investigated yet, the global mean value (Murray et al., 2011) is used for the calculation.
Location | Area (ha) | Annual carbon burial rate (t CO2 e ha−1 yr−1) (a) | Soil organic carbon (t CO2 e ha−1) (b) |
---|---|---|---|
Ganghwa tidal flat | 35,300 | 8.0±8.5 | 349.20 |
Source: Global average and standard deviation of carbon sequestration rate for salt marshes (Murray et al., 2011).
The potential monetary value of blue carbon is the ‘CO2 reduction (emission avoidance + annual new sequestration)’ multiplied by the price received per unit of reductions. The ‘CO2 reduction’ is the quantity of CO2 e, of which release would be averted by avoiding conversion of tidal flats and influx of which would be stored in the soil by burial (Murray et al., 2011). Some studies show that methane (CH4) and nitrous oxide (N2O) emissions can be ignored in areas affected by tides (Chmura et al., 2003). Therefore, CO2 e caused by CH4 and N2O were excluded from the calculation. Using the following equation, the ‘CO2 reduction’ is monetized by multiplying the annual CO2 e reduction by a stream of expected carbon prices over time horizon of t. All calculated values are real values without inflation.
where, r: social discount rate (2%, 4.5%, 7%)
t: time horizon (25 years)
According to Bu et al. (2015), soil respiration is three times higher in reclaimed lands than in salt marshes. After reclamation, therefore, the previously sequestered soil organic carbon pool may decline to 50% in approximately 10 years (Bu et al., 2015). This release is based on the assumption that only the top meter of soil is disturbed and reflects an exponential decay function whereby soil organic carbon has a half-life of 10 years. Decay of the remaining biomass carbon is much slower after the half-life, and 8% of the soil organic carbon remains at the end of the 25-year period (Murray et al., 2011). Based on a 10-year half-life and the 25-year of 8% residual (red line in Figure 2), an exponential emission curve from the disturbed or converted Ganghwa tidal flat was drawn (blue line in Figure 2), and the annual amount of release of carbon was estimated (Table 5) from the following equation of this curve.
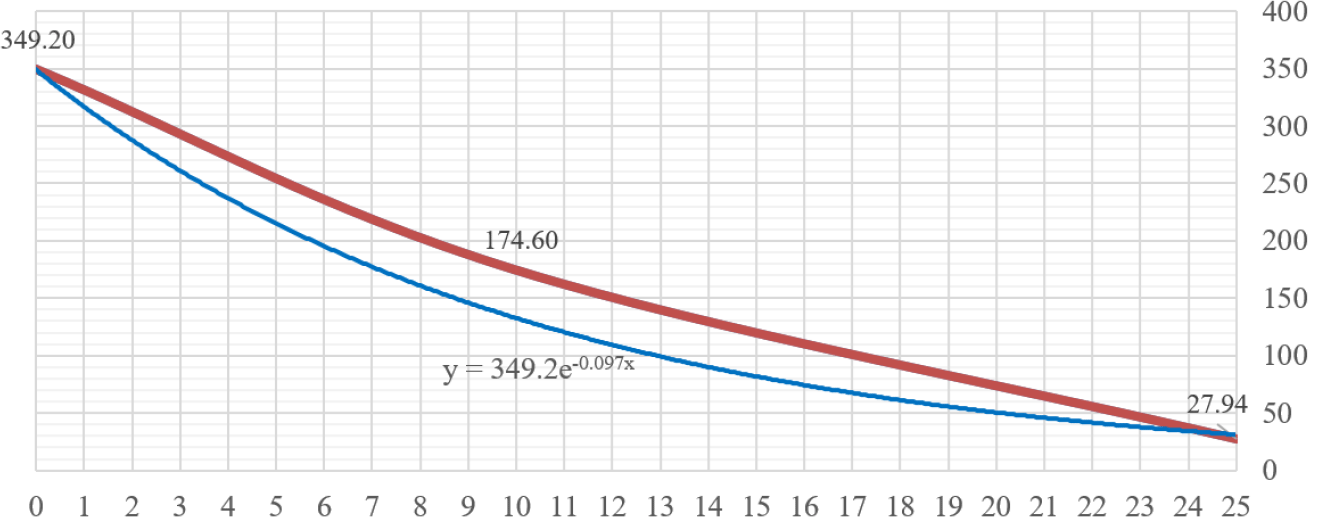
where, y: residual soil organic carbon (t CO2 e/ha)
x: elapsed years after disturbance or conversion (yrs)
Table 5 shows the accumulated amount of carbon emission with elapsed years after the disturbance. These values are obtained by subtracting the amount of soil organic carbon remaining after disturbance (y in the above equation) from 349.2 t CO2 e/ha which is the amount before disturbance. Table 6 presents the total of prevented emissions each year due to not disturbing the tidal flat, and the total newly sequestered by the tidal flat each year.
To estimate a monetary value of blue carbon, the amount of avoided carbon emissions and newly sequestrated carbon in the Ganghwa tidal flat are calculated first (Table 6), and then discounted back to the present with a 4.5% discount rate. The carbon price range used is $5–30 /t CO2 e, taking into account current carbon prices in compliance and voluntary markets across the world (Table 7).
Markets | Price / t CO2 e | Source | Date for price | ||
---|---|---|---|---|---|
EU ETS | EUA (EU emission allowances) | €21.40 | US$24.02 | EEX (https://www.eex.com) | 04/02/19 |
CA-ETS | California carbon allowance | US$15.10 | California carbon dashboard (http://calcarbondash.org) | 03/29/19 | |
ACCU | Australia offset prices | A$15.72 | US$11.19 | Carbon pulse (https://carbon-pulse.com) | 02/04/19 |
NZU | New Zealand prices | NZ$25.70 | US$17.52 | Carbon pulse | 02/04/19 |
KAU | Korean allowance unit | ₩26,650 | US$23.52 | Carbon pulse | 02/04/19 |
KOC | Korean offset credit | ₩26,500 | US$23.39 | Carbon pulse | 02/04/19 |
Voluntary market | Forestry and land use | US$5.1 | Forest trends (a) (https://www.forest-trends.org) | Average price in 2016 |
Source: Hamrick and Gallant, 2017.
The PV of ‘CO2 reduction’ in the Ganghwa tidal flat is 245 million dollars with a discount rate of 4.5% and the carbon price of $20 /t CO2 e that is similar to the carbon market price in Korea as of April 2, 2019 ($23.52). Under the same circumstances, the total monetary value of coastal carbon stored in Korea’s tidal flats is estimated at $1.276 billion (~1.5 trillion won) (Table 8).
4. Cost-Benefit for Tidal Flat Conservation
To assess the costs associated with conservation measures for tidal flats, two strategies are considered: protection from conversion; and restoration of coastal wetland. Tidal flats can be protected from conversion to other uses through the establishment and management of Coastal Wetland Protected Areas (CWPA). Restoration refers to the introduction of vegetation in unvegetated areas to increase carbon stocks. For the benefit, the PV (emissions avoidance + annual new sequestration) estimated in the section above is regarded as the benefit of the protected area establishment, and the value of the additional carbon stored by the coastal wetland restoration is calculated as the benefit of restoration.
Costs associated with establishing and managing CWPA are divided into direct and indirect costs. Direct costs generally include administrative activities. Indirect costs may include the opportunity costs from the establishment of a no-take zone, which prohibits any activity such as fishing and mining, and other opportunity costs for development lost due to the establishment of CWPA. However, this report only evaluates direct costs for the following reasons. Korea’s CWPA allows fishing to continue where the local fishing communities have traditionally cultivated, captured, or harvested for the purpose of livelihood or recreation, so the establishment of a CWPA may not involve the creation of a no-take zone. In addition, the purpose of this paper is not to analyze the cost-benefits on a specific development, but to assess the benefits of carbon stored in tidal flats and to estimate the costs of preserving these values.
To estimate the direct costs of CWPA establishment and management, the Ministry of Oceans and Fisheries’ internal 2008–2012 budget data (Son et al., 2013) can be used. This sets the cost for CWPA establishment at $263,469 yr−1 (price levels adjusted to reflect 2019 money value), which consists mostly of administrative expenses for hearing from stakeholders and local governments, consultation of experts and scientists, and evaluation processes. Given that, on average, one CWPA is designated per year in Korea, and that the CWPA establishment cost does not vary greatly between areas, this figure ($263,469) can be used more generally as the CWPA establishment cost. Note that the establishment cost occurs at the time that the CWPA is designated. According to the same budget data, the CWPA annual management cost is $2,160,448 yr−1 (price levels adjusted to reflect 2019 money value), which is mostly composed of monitoring, outreach and education expenses. Using this data, the cost per unit area is calculated as $99 ha−1 yr−1 (price levels adjusted to reflect 2019 money value), which leads to $1,469 ha−1 over 25 years with a 4.5% discount rate (Table 9). (The conversion of value from 2010 to 2019 is based on the inflation rate of 1.96% over the past decade in Korea [K indicator, 2019]). So, if Ganghwa tidal flat was designated as a CWPA, the total cost for CWPA establishment and management would be estimated at $52 million with a 4.5% discount rate.
For the purpose of CWPA establishment and management, the PV of ‘CO2 reduction’ (emission avoidance + annual new sequestration) estimated in the previous section is used. The benefit of ‘CO2 reduction’ for the Ganghwa tidal flat is $61–367 million with a discount rate of 4.5%, when the carbon price is $5–30/ t CO2 e (Table 8). The Ganghwa tidal flat is under high pressure for development, so if it is developed, this benefit will be converted into an opportunity cost.
If a tidal flat that stores large amounts of carbon is designated as a CWPA, coastal wetland restoration–introduction of vegetation in unvegetated tidal flats–may be considered as an enhancement of the carbon sequestration service. In fact, seven tidal flat restoration projects have been implemented in Korea since 2010. The cost of this restoration varies greatly. The economies of scale are evident (Figure 3) and the costs vary depending on site conditions and restoration methods. The average cost for coastal wetland restoration is estimated at $97,663 ha−1. This includes the planning and construction costs. Planning costs include various administrative procedures, environmental impact assessment (EIA) and feasibility studies. Construction costs may include land acquisition or compensation for fisheries. Given that planning costs generally account for about 10% of total restoration costs, the cost is divided between $9,770 ha−1 for planning and $87,890 ha−1 for construction.
The ‘economy of scale’ is expressed in equation (1), such that the larger the restoration area, the smaller the restoration cost per unit area.
where, y: coastal wetland restoration cost ($/ha)
x: project scale (ha)
To apply this calculation, the cost per unit area of restoration (marginal cost) is calculated (Figure 4). The marginal cost decreases steeply until the restoration scale of 5% is reached, but little after that. However, the restoration scale cannot be greatly increased, partly because the widespread distribution of fishing rights in Korea’s tidal flats is a major factor limiting the scale of coastal wetland restoration. Therefore, it is assumed that only the upper 10% of the intertidal zone is restored in consideration of the fact that fishing is mainly performed in the middle and lower parts of tidal flats.
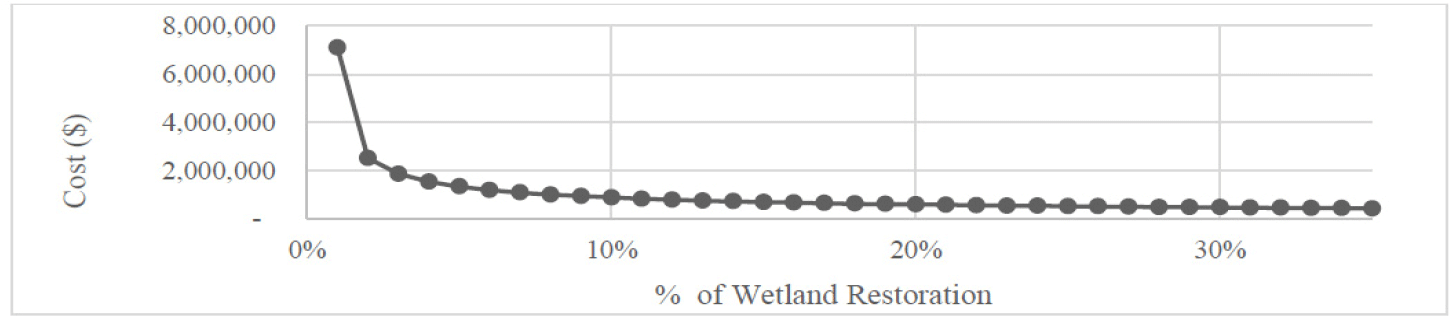
Considering that the ‘global average and standard deviation of carbon sequestration rate for salt marshes’ is 8.0 8.5 t CO2 e ha−1 yr−1 (Murray et al., 2011), the sequestration rate of carbon additionally stored through wetland restoration is assumed to be 8 t CO2 e ha−1 yr−1. This means that carbon is now being stored at the rate of 8 t CO2 e ha−1 yr−1, and 8 t CO2 e ha−1 yr−1 carbon additionally will be stored through wetland restoration. The higher value of the standard deviation is adopted as it is assumed that the restoration and thorough management can maximize the carbon storage capacity.
When 10% of the Ganghwa tidal flat is restored, the cost is calculated at $19 million (Table 10), and the benefit from additionally stored carbon through wetland restoration is estimated at $2–13 million with a discount rate of 4.5% and a carbon price of $5–30/ t CO2 e (Table 11). In this calculation, the cost is higher than the profit due to the initial high construction cost. It should be noted the net benefit of restoration occurs over a long period of time, of more than 25 years.
Location | Area restored (ha) | Cost | |
---|---|---|---|
($/ha, one-time) | ($, one-time) | ||
Ganghwa tidal flat | 3,530 | 5,486 | 19,365,580 |
However, to attract investment from private sectors, efforts are needed to lower the construction costs ($19 million).
The net benefit is estimated assuming that the Ganghwa tidal flat is designated as a CWPA and the wetland restoration project is implemented in 10% of the total area. It is estimated to be $182 million at a 4.5% discount rate and with $20 /t CO2 e of carbon market price (Table 12).
The net benefit is highly variable, depending on the carbon market price and the discount rate. If the carbon price is $5 and 10% of the Ganghwa tidal flat is restored, the net benefit is $–8.2 million. However, as carbon prices rise, wetland restoration projects become more economically feasible. At a discount rate of 4.5%, the break-even point between benefit and cost occurs when the price of carbon is $4–6 /t CO2 e (Figure 5). Here, the lowest value of the range (4 t CO2 e) is the case when the tidal flat stays intact without restoration, whereas the highest value of the range (6 t CO2 e) is the case when 10% of the tidal flat is restored. Given that the average carbon market price in 2018 in Korea is $20.62 /t CO2 e, the concept of blue carbon is economically viable (Figure 6).
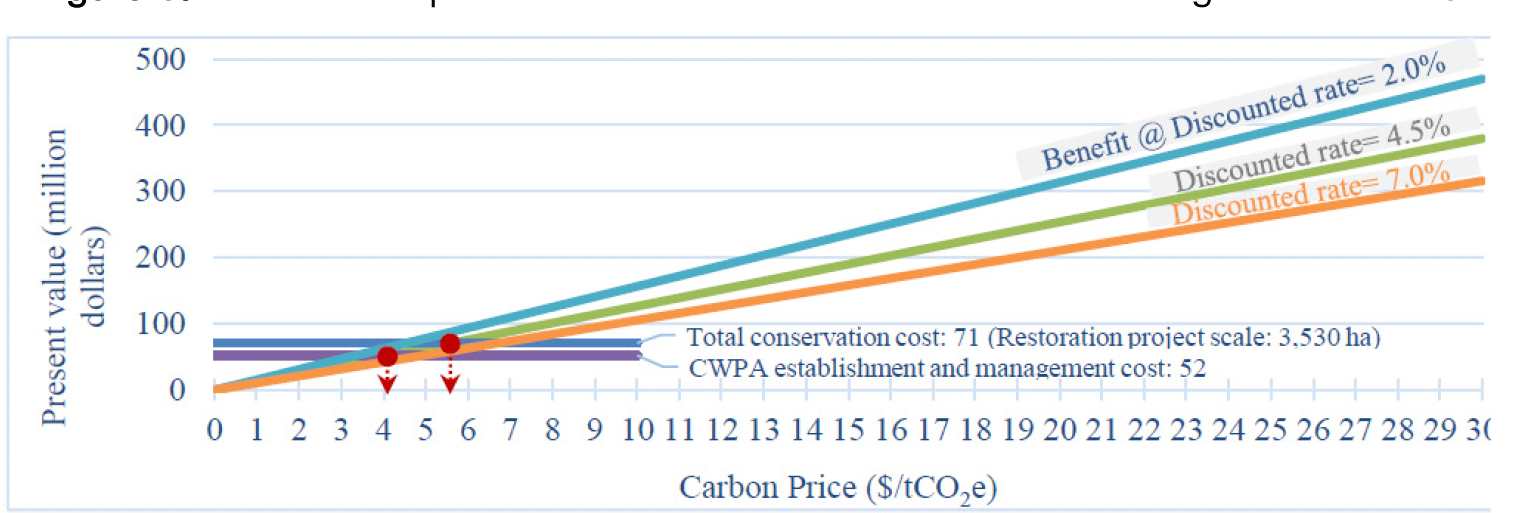
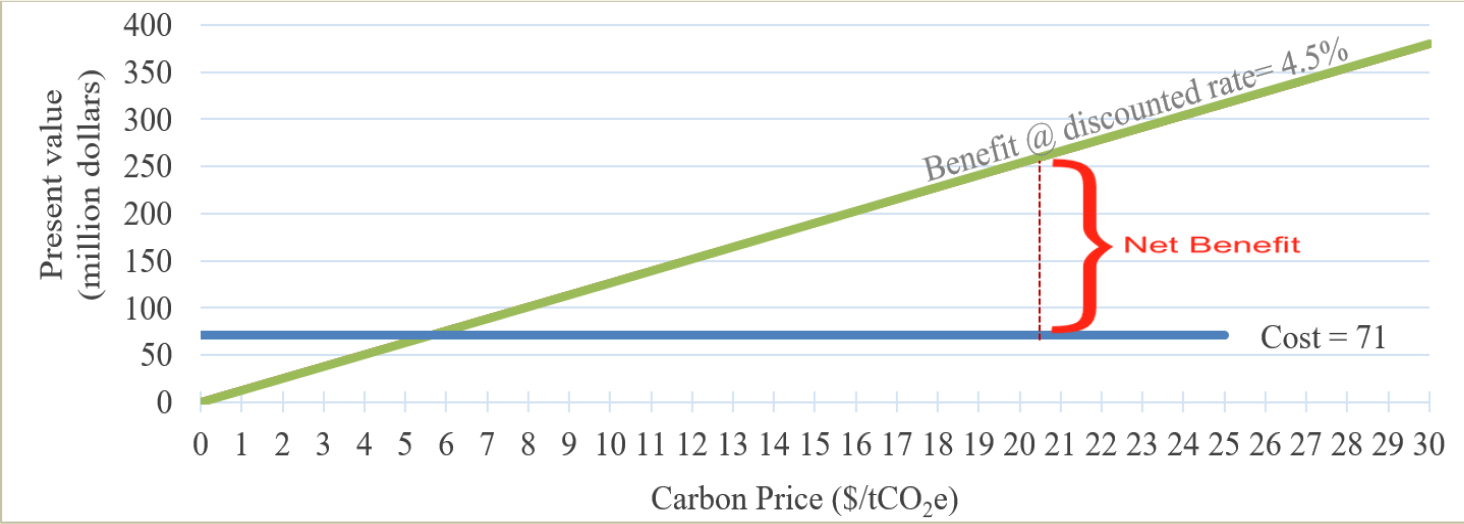
This net benefit is estimated only taking into account the carbon storage service among the many benefits provided by tidal flats. Additional benefits comprise other market benefits (use values or direct use values) and non-market benefits (non-use values or in-direct use values). Use values include direct enjoyment or consumption of environmental goods such as fishing and tourism, and non-use values include the benefits of conserving resources for future generations and the joy of knowing that something exists (Keohane and Olmstead, 2007). Including these additional use and non-use values would result in greater net benefits and lower break-even points between benefits and costs. It is therefore important to ensure these other benefits are not overlooked in decision-making processes for coastal development.
The previous calculations are based on the “market value” of carbon. There is another important value, the “Social Cost of Carbon (SCC).” This is the cost of the long-term economic damage caused by a ton of carbon dioxide, and thus the cost to society. The US government set a country-level SCC of $42 per t CO2 for 2020 at a 3% discount rate (EPA, 2019). Science suggests that this cost is probably much higher. Recently Ricke et al. (2018) estimated that the median value of the global SCC (GSCC) is $417 per t CO2 (66% confidence intervals, range: $177–805 per t CO2). When GSCC is applied, the net benefit for the Ganghwa tidal flat is $5,204.8 million (Table 13).
The application of the GSCC shows a considerably higher net benefit even if the conservation activities of the tidal flats do not consider other ecosystem goods and services. However, even though the “market price” is applied, the conservation policy is still worthy in the sense of economic gain, as shown in Table 12.
5. Conclusions and Policy Implications
Considering the market transaction possibility of coastal blue carbon, it is important to mitigate market failure by calculating its market value. To estimate the net benefit of the tidal flat conservation, cost-benefit analysis was carried out using the 2018 nation-wide blue carbon survey results. With a 25-year time horizon, the break-even point between benefit and cost of the Ganghwa tidal flat occurs when the carbon price is $4–6 /t CO2 e. Given that the average carbon market price in 2018 in Korea is $20.62 /t CO2 e, the ‘blue carbon’ valuation is high enough to incentivize coastal wetlands conservation, and climate change mitigation and adaptation policies.
Some legislative improvements and future considerations are required to reflect blue carbon in the policies for tidal flats conservation and climate change mitigation and adaptation. Legislative improvements for existing laws could involve:
Designating protected areas (CWPA, MPA) in areas where carbon is stored in large amounts, setting basic principle to reflect climate change mitigation and adaptation technologies in coastal management projects, and including cost-benefit analysis–with the monetary values of blue carbon and other goods and services ecosystem–in the ‘Basic Plan for Reclamation.’
Successful inclusion of blue carbon in the carbon offset program could increase participation of the private sector. In this regard, there are two things to consider: financing for market transaction and allocating use rights. First, market transaction and information necessary for market trading involves high costs. Government can play a key role in eliminating some of the barriers that lead to high transaction and information costs. To prepare for market transactions, the Korean government needs to carefully consider its role, institutional arrangements for cost saving, and approaches to encourage intermediaries and private participation. Second, when a use right or management right is allocated to the private sector for coastal wetland restoration, it needs to be clearly set out the principles and guidelines on type of management effort (e.g., restriction of fishing activities, labor input), distribution of benefit, and specific guidelines for use consistent with the public interest. Clearly stated principles and guidelines can help prevent possible conflicts that may arise between the governments, beneficiaries (users) and fishing communities.
Currently, the most important barriers to the introduction of blue carbon policy in Korea are the lack of understanding of blue carbon, lack of involvement in the climate-related policy mechanisms, and low preference for carbon offsetting (referring to forest carbon). To encourage the use of blue carbon in tidal flat conservation and climate mitigation and adaptation policies, it is necessary to assess the variance of carbon stocks in tidal flats, participate in climate-related government and international networks, and conduct more research to examine market trading methods and possibilities by referring to Korean forest carbon offset scheme.
Blue carbon is economically important and can be used in various coastal conservation and management policies. The Korean government should actively support to achieve meaningful achievements of the blue carbon policy. This can lead to a virtuous cycle structure in which a cap-and-trade secondary market price could incentivize blue carbon. This could be a significant contribution to a national target for carbon reduction and target 13.2 of the United Nation’s Sustainable Development Goals.